Collaboration of hybrid quantum computers
Vision and goals
Basic and applied research in quantum technology, with direct applications in communications, sensing, and information processing, is a compelling area of research for both academia and industry. Research in quantum technology today will help define the 21st century in ways similar to what the transistor achieved in the 20th century, enabling our modern-day electronic, computing, and networking revolution. Anticipating the quantum revolution, numerous international research groups in different disciplines are currently developing architectures using photons, ions, neutral atoms, superconductors, and optomechanical devices to achieve this goal. Trapped ions have been at the forefront of quantum information processing for decades. They feature high quantum gate fidelity (99.97%), long quantum information storage time (minutes or beyond), and efficient remote entanglement and teleportation. A prototype of a universal quantum computer with 32 qubits, minimal gate errors, and a world-leading quantum volume is expected to be available on the cloud this year by a new emerging technology company, IonQ. In parallel with the development of ion trap quantum computers, superconducting circuit platforms, with architectures resembling classical computers based on semiconductor devices, are being pursued by long-established technology companies, such as Google, IBM, and Intel. Today, superconducting circuit quantum computers implement more qubits (beyond 100 in the near future), offer three orders of magnitude faster quantum gates, and allow more integrated (laser-free) constructions. However, due to the lack of reliable mechanisms to store and teleport quantum information, excess quantum and classical information conversions must be performed in solving complicated problems. This limits the design and scope of quantum algorithms.
In the pursuit of optimal quantum processing, storage, and teleportation, a dedicated quantum hybrid system (i.e., integrating architectures) will perform fast information processing encoded in superconducting circuit qubits and store and teleport the processed information in the form of trapped ion qubits. A significant challenge in realizing such a hybrid system is the ability to translate information between the different qubit types coherently. Due to the substantial difference in mass and dimension, direct coupling between the macroscopic superconducting circuits and a single ground state ion is either slow or far off-resonance. Several approaches, such as direct electron motional coupling, ion motional coupling with frequency modulation, and molecular rotational coupling, have been proposed as quantum interconnects.
Our team propose a novel atom-based and chip-scale quantum transducer, exploiting a Rydberg atom to facilitate efficient quantum state transfer from a superconducting circuit to a long-lived spin state of an atomic ion, processes (1) and (2). Based on numerical calculations, we estimate a unity transducing efficiency of a single qubit is expected, and scalability up to hundreds of qubits is realizable. The proposed experiment is a major building block to enable the construction of a universal quantum computer with hybrid architecture. The achievement of our goals will lift investigations of quantum computation to the next level, where gate speed, fidelity, memory time, and scalability would be simultaneously superior. Success in our project goals will facilitate the next generation of quantum computers beyond the Noisy Intermediate Scale Quantum (NISQ) paradigm.
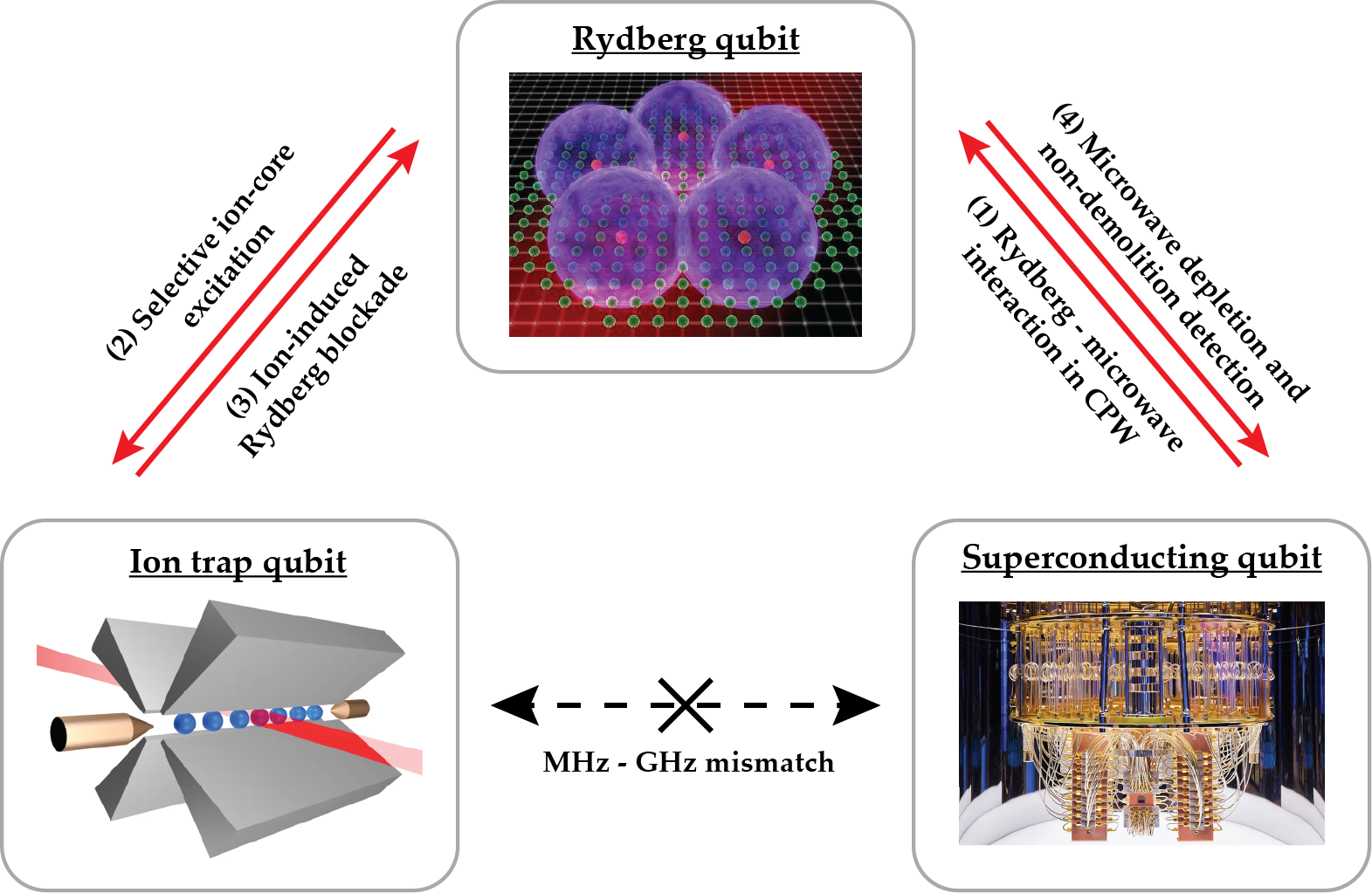
Our team
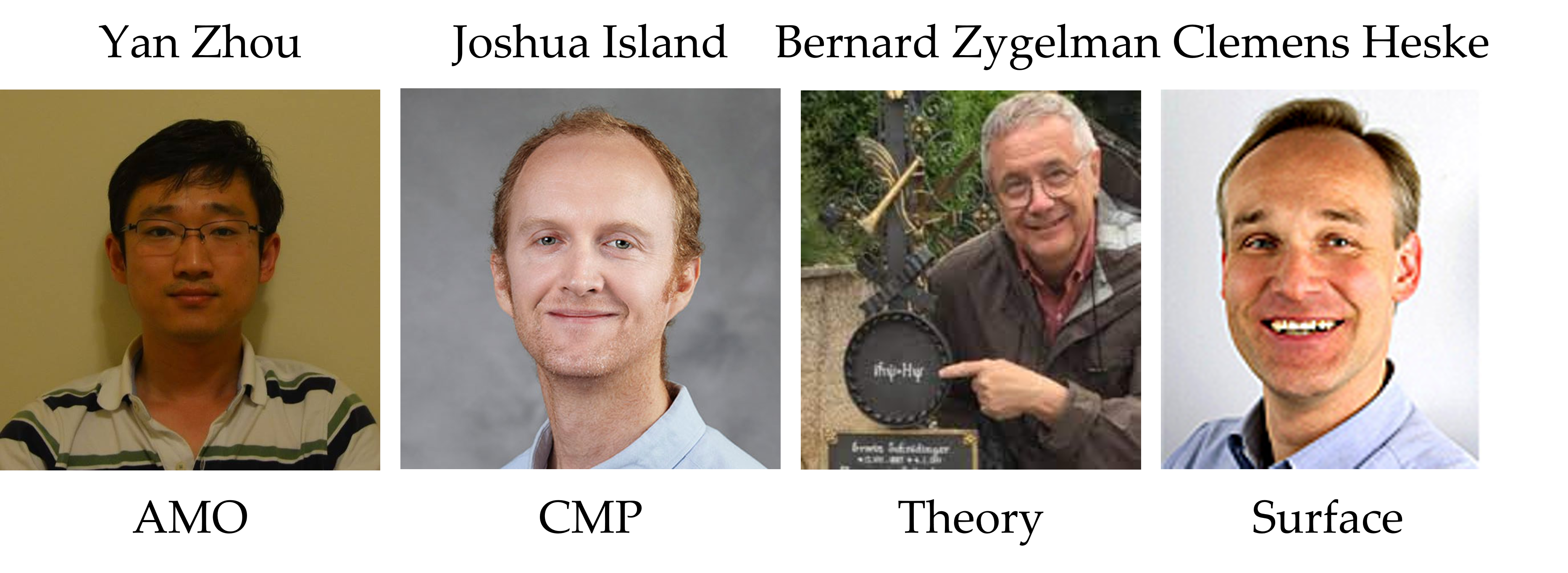
Approach and methodology
The kernel of our approach is the application of the circular Rydberg state of strontium (Sr) atom, which is hypersensitive to a microwave field and has a long lifetime. The long-lived circular state supports a design that separates the entire setup into five distinct spatial zones with inertial or optical navigation of the atom across them. This unique arrangement can effectively eliminate crosstalk, such as scattered photons from the atomic source and electric noise from the ion trap. Unlike other quantum transducer proposals that yield an unstable qubit, such as a Rydberg qubit or photon, we propose a novel method to coherently stabilize the Rydberg qubit into the spin state of an atomic ion using selective ion-core excitation. Next, a standard quantum logic protocol can be applied to transfer the information from a spin-state to a motional state for further quantum information processing in a standard ion trap quantum computer.
The top panel shows the critical experimental layout, and the bottom panel shows the quantum state evolution in our proposed experimental sequence. Our quantum state transfer algorithm begins with a single Sr atom that is cooled, trapped, and loaded into an optical tweezer (I). A laser pulse then pushes the atom toward an on-chip coplanar waveguide resonator (CPW) (III). When the Sr atom is midway (II) between the tweezer and the CPW, two laser pulses excite it to the 68d, m = 2 Rydberg state (a). Immediately after the laser excitation, a clockwise (σ+) RF field coherently transfers the Sr atom to a circular state l = m = n-1 = 67 (b). Next, the Sr atom travels across the CPW at a 30 µm altitude (III). Simultaneously, a transmon qubit, fabricated on the same chip as the quantum bus, is operated in the dispersive limit, detuned from the CPW. Quantum state transfer occurs with the interaction between a single microwave photon trapped in the CPW and a Rydberg state of Sr atom with a large transition dipole moment (c). The microwave photon is expected to be transferred coherently from the CPW to the Rydberg state in 300 ns, significantly quicker than the CPW lifetime (5 µs) and atom transit time (5 µs). When the Sr atom travels to the ion qubit stabilization station (IV), opposite to the circularization process, a counter-clockwise (σ-) RF field de-circularizes the circular state n = 68, l = 67 to a low-l state (d). However, this de-circularization field is invalid for n = 67, l = 66 because of a non-resonant detuning. Therefore, if the Sr atom has absorbed the microwave photon, it is kept in a circular state. Otherwise, the Sr atom is transferred to a low-l state. Immediately after the de-circularization transfer, a laser is applied to excite a narrow Sr+ ion-core transition (e). A laser tuned for the ion-core excitation of the circular Rydberg state is off-resonant for that of the low-l Rydberg state. Therefore, the Sr+ ion-core of the Rydberg state that has or has not absorbed the microwave photon is in the metastable 2D3/2 state or the ground 2S1/2 state, respectively. Finally, a half-cycle THz pulse (500 V/cm) is applied to remove the Rydberg electron to create a Sr+ ion that is stopped and sent to an ion trap (V). In this way, we coherently transfer the single microwave photon generated by the superconducting circuit to the long-lived ion qubit.
In addition to the transduction from the superconducting circuit to ion qubit, a reverse process that coherently transfers an ion qubit state to a superconducting qubit state could be achieved using a similar scheme. First, a controlled long-range interaction between the ion and a Rydberg atom transfers a motional ion qubit to a Rydberg qubit. Large polarizability of the Rydberg atom supports a strong long-range interaction that eliminates micromotion-induced incoherent ion-atom collisions. Theoretical calculation shows a >99% fidelity can be achieved. Then, the Rydberg atom travels across the CPW to deplete one microwave photon. This depleted microwave photon is injected into the CPW from a Fock-state photon source without carrying any quantum information. The quantum information is encoded in the status of depletion that is determined by the Rydberg qubit. Finally, a quantum non-demolition detection maps the microwave photon number in the CPW onto a qubit state in a single-shot by means of qubit–photon logic gates.
