Exploring new physics beyond the Standard Model
Motivations
The Standard Model of particle physics serves as a milestone in describing the fundamental constituents of matter and their interactions. However, it appears to be an incomplete theory raising many unanswered questions, such as what dark matter and dark energy are, what mechanism induces matter and anti-matter imbalance, and why the strong interactions do not exhibit significant Charge and Parity (CP) violation. One of the ways to test the Standard Model is to probe and explore new particles created in a high-energy collider. An alternative method is to sense the forces and interactions generated by the new particles using extremely sensitive quantum sensors, such as polar molecules. In this proposal, we propose to build a single experimental setup but explore multiple new physics in different territories, from particle physics and nuclear physics to cosmology, such as: (1) examination of Time-reversal and Parity (T,P)-violated symmetries in the leptonic sector of the Standard Model by probing the electron’s Electric Dipole Moment (eEDM); (2) examination of T,P-violated symmetries in the hadronic sector of the Standard Model by probing the Nuclear Magnetic Quadrupole Moment (NMQM); (3) investigation of spin-dependent parity-nonconservation (SD-PNC) effects in the nucleus by measuring the Neutron Quadrupole Moment of Nucleus (NQMN); (4) searching for the axion–a cold dark matter candidate–by detecting oscillations of eEDM and NMQM.
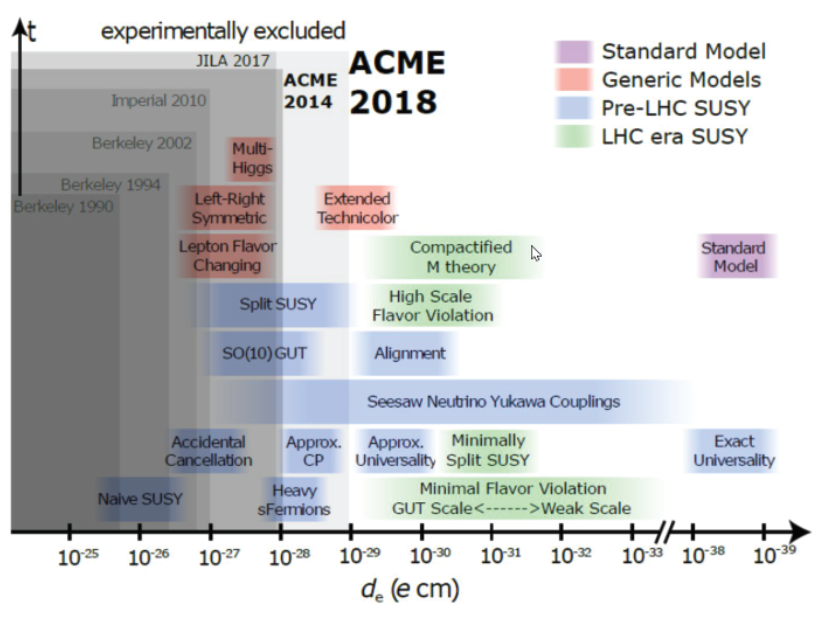
Figure 1. Experimental progress all over the word. The figure is complied by Dr. David DeMille. The best eEDM measurement performed by ACME in 2018 has reached an equivalent energy of 50 TeV that is 10 times more than LHC
Methodologies
Goals
Ongoing progress
Collaborations
We are constructing a novel experimental platform (Figure 2) with a combination of quantum logic technology, 2D ion trap arrays, and integrated photonic devices to perform precision measurements of polar molecular ions. The quantum logic scheme is applied to control and readout the quantum states of molecular ions with near-unity fidelities. The 2D ion trap array will increase the experimental throughput and thus increase measurement accuracy. An integrated photonic device will be used for highly efficient and scalable light delivery and signal detection. To induce tenth of GV/cm effective electric field, about 10 V/cm rotating bias electric field is applied via a centrifugal force in the lowest rotational normal mode of the trap, which is sufficient to completely polarize molecules with small Ω-doubling structures. An effective magnetic field that is synchronized to the rotating electric field could be generated either by modulated Berry’s phase or RF fields. To reduce the decoherence from blackbody radiation and stray magnetic field, the 2D ion trap array is fabricated on a 1 inch by 1 inch chip and housed in a 4K cryogenic enclosure with multi-layer mu-metal magnetic shielding.
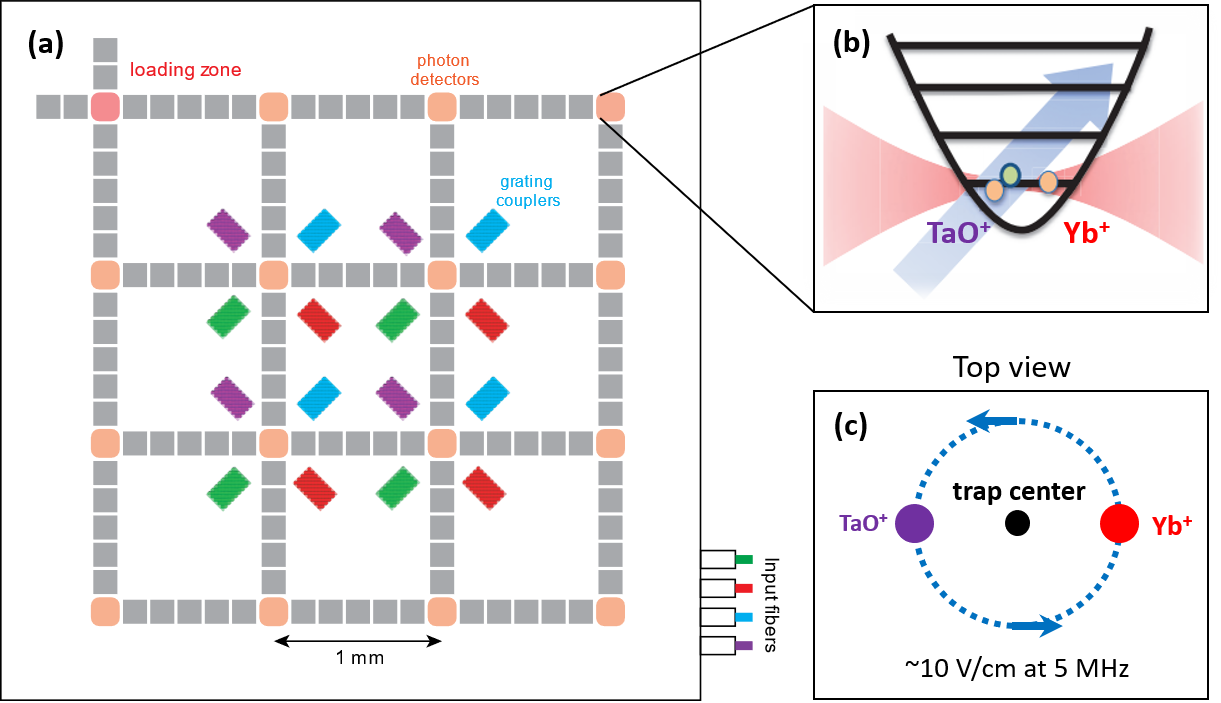
Figure 2. (a) Precision measurements of molecular ions using a 2D surface trap array and integrated photonic device for light delivery and detection. (b) Performing state control and readout with high fidelity by the quantum logic scheme. (c) Generating a rotating bias electric field by a centrifugal potential in the lowest rotational mode.
Molecular choice
We choose 181TaO+ because: (1) TaO+ is an ion species that can be trapped for a long time; (2) the T,P-odd sensitive state, 3Δ1 of TaO+, is predicted to be the ground state with a small g-factor, which facilitates a long coherence time; (3) TaO+ has a relatively large effective electric field (20.2 GV/cm) that is sensitive to T,P-odd eEDM and nucleon-electron scalar-pseudoscalar (SPS) interaction; (4) 181Ta has an I=7/2 nuclear spin and a possibly deformed nuclear structure that provides a large enhancement of the T,P-odd NMQM and SD-PNC NQMN; (5) 181Ta is a stable isotope and has ∼100% natural abundance; (6) 16O does not have a nuclear spin, which simplifies the molecular hyperfine structures. Table 1 compares sensitivities of TaO+ and ThF+ for varieties of fundamental parameters. ThF+ is under active study towards improving the current best measurement of eEDM by one or two orders of magnitude at the Joint Institute for Laboratory Astrophysics (JILA) at the University of Colorado Boulder. Although ThF+ has a factor of two better sensitivity for the eEDM, TaO+ can be applied to probe NMQM that could reveal new physics in the hadronic sector of the Standard Model as well.
Table 1. Theoretical fundamental interaction constants of 181TaO+ and 232ThF+, and the current best experimental constraints for the eEDM and the NMQM. The NMQM could be produced by the proton EDM dp, the QCD θ term, and the difference of the quark chromo-EDMs (du-dd). The values in the last four columns are equivalent to experimental frequency accuracies in µHz.
Molecule | I | Eeff | WSP | |WddeS| | |WMMS| | ||
---|---|---|---|---|---|---|---|
GV/cm | kHz | de=10-29e.cm | dp=10-25e.cm | θ=1×10-10 | du-dd=10-27e.cm | ||
181TaO+ | 7/2 | 20.2 | 17.7 | 49 | 13 | 21 | 13 |
232ThF+ | 0 | 35.2 | 50 | 85 | - | - | - |
173YbF | 5/2 | 14.5 | 40.5 | 35 | 22 | 42 | 25 |
232ThO | 0 | 81.5 | 112 | 200 | - | - | - |
Measurement scheme
We focus primarily on measuring static T,P-odd effects. Information from the leptonic and hadronic sectors of the Standard Model can be extracted from a single measurement scheme as described in Figure 3. Our experiment inherits a general measurement scheme from eEDM experiments using HfF+ and ThF+ ions in a rotating frame that are in active study at JILA. There are more benefits in the TaO+ experiment. First, TaO+ has more electron spin resonances with the same T,P-odd interaction but different magnetic field susceptibilities, which further suppresses the magnetic field induced systematics. T,P-odd interactions can be extracted from a linear combination of more than two of such frequency measurements. Second, different hyperfine states of TaO+ have the same eEDM and nuclear-electron SPS interaction but different NMQM sensitivities. Thus, we can separate the T,P-odd interactions in the leptonic sector from those in the hadronic sector by performing measurements in different hyperfine states, which is descried by the equations shown in the Figure 3. Last, the process of data collection will include accurate time stamps to each measurement for further time-dependent analysis, which could reveal evidence of the axion. It is a bonus to perform investigations in cosmology without additional experimental efforts.
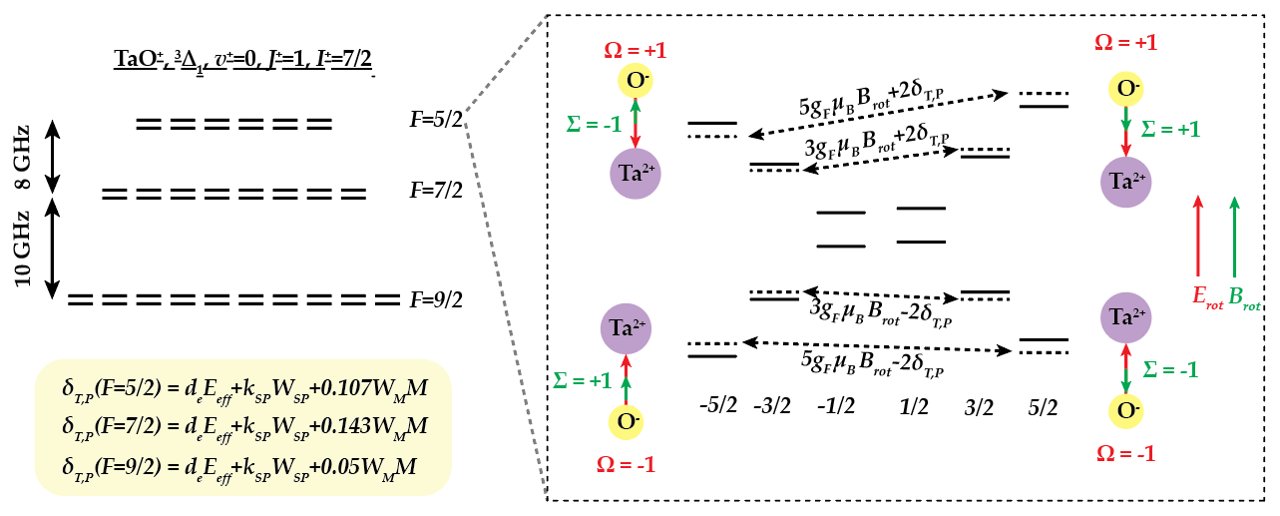
Figure 3. Measurement scheme of the eEDM and the NMQM using ultracold TaO+ molecular ions. Left figure shows hyperfine structures of 181TaO+, 3Δ1, v+=0, J+=1, I+=7/2 without applied electric or magnetic fields. For each group of hyperfine states with a specific F quantum number, there are 2(2F+1) magnetic sub-levels with Ω-doubling structures of opposite parities. Right figure shows quantum states of F=5/2 with applied electric and magnetic fields as solid lines. Dashed lines represent state shifts induced by T,P-odd interactions. Dashed arrow lines show actual frequency measurements, which contain information of both T,P-even electron spin resonance and T,P-odd interactions. Different categories of T,P-odd interactions (de and kSP vs. M) can be extracted by performing measurements in different hyperfine states. de is the eEDM, kSP is the nuclear-electron SPS, and M is the NMQM. Eeff, kSP and WM are parameters connecting T,P-odd effects to actual frequency shifts. Such parameters are obtained from calculations of molecular electronic structures.
Target sensitivity
The PI was previously a research associate in the JILA eEDM research group and participated in three generations of eEDM measurements using trapped molecular ions. A direct comparison between the proposed quantum logic ion experiment at UNLV and the ongoing bucket brigade ion experiment at JILA is listed in Table 2. Although both experiments use ground state molecular ions, their experimental architectures are quite different. The JILA experiment is superior at having a large total ion number. The UNLV experiment aims to increase the state preparation and readout efficiency. Furthermore, the compaction of the chip trap that facilitates more accessible blackbody radiation and magnetic shielding, the few high-power pulsed lasers involved, and the single ion (or dual ion including logical ion) per trap site will be beneficial for better coherence, smaller systematics, and longer data collection duration (invulnerable to equipment failure). In all, UNLV experiment expects a comparable accuracy of the frequency measurements to the JILA experiment.
Parameters | UNLV TaO+ | JILA ThF+ |
---|---|---|
TaO+ or ThF+ ion number per site | 1 | 3000 |
Number of trap sites | 100 | 50 |
State preparation efficiency | 90% | 40% |
State readout efficiency | 90% | 20% |
Contrast | 1 | 0.6 |
Coherence time | 100 s | 20 s |
Experimental duty cycle | 90% | 90% |
Data collection duration | 1000 hours | 300 hours |
Frequency accuracy | 0.5 µHz | 0.3 µHz |
Current progress
under construction